Introduction
Laser beam homogenization is a pivotal technique in laser optics that aims to improve the uniformity and consistency of laser beams. In many laser applications, such as material processing, lithography, and medical treatments, the spatial intensity distribution of the laser beam plays a crucial role in determining the quality and precision of the results.
However, due to factors like thermal gradients, optical imperfections, and diffraction effects, laser beams often exhibit non-uniformities in their intensity profiles, leading to undesirable effects like hot spots or irregularities in the processed material.
To mitigate these issues, laser beam homogenization techniques are employed to transform the input laser beam into a more uniform intensity distribution across its cross-section. This process involves various methods and optical components designed to reshape the beam profile while preserving its coherence and other essential characteristics.
By achieving a more uniform intensity profile, laser beam homogenization enhances the precision, consistency, and efficiency of laser-based processes, ultimately enabling better control and quality in a wide range of applications. From industrial manufacturing to scientific research, the significance of laser beam homogenization cannot be overstated, as it unlocks new possibilities and advancements in diverse fields reliant on laser technology.
How do Laser Homogenizers work?
Laser beam homogenizers employ a range of techniques to transform the input laser beam into a more uniform intensity distribution. One common method involves the use of diffractive optical elements (DOEs) or refractive beam shapers.
These elements redistribute the energy of the incoming laser beam, effectively smoothing out variations in intensity across its cross-section. By carefully designing the surface profile of the DOE or the shape of the refractive optics, specific spatial intensity patterns can be generated, such as flat-top or Gaussian profiles, tailored to the requirements of the application.
Another approach to laser beam homogenization is through the use of fly’s eye lens arrays or microlens arrays. These arrays consist of multiple small lenses arranged in a grid pattern. Each lens focuses a portion of the incoming laser beam onto a different point in space, and when combined, these individual beams overlap to create a more uniform intensity distribution.
The spacing and size of the lenses determine the degree of homogenization achieved, with denser arrays providing finer control over the beam profile.
Furthermore, techniques like beam scanning or spatial filtering can also contribute to laser beam homogenization. Beam scanning involves moving the laser beam across the target surface in a controlled manner, averaging out any intensity variations over the scanned area. Spatial filtering utilizes optical elements to selectively remove high-frequency components or irregularities from the beam, resulting in a smoother intensity profile.
Types of Laser Beam Homogenizers
There are two widely used methods of laser beam homogenization for systems where uniform beam profiles are needed: homogenization using a lens array optic and homogenization using a light pipe rod.
Each method has its own advantages and disadvantages. We will be describing those in this post along with principals of operation.
Lens array homogenizers
A lens array is composed of separate lens elements stacked side by side to form an array then placed in the laser beam path.
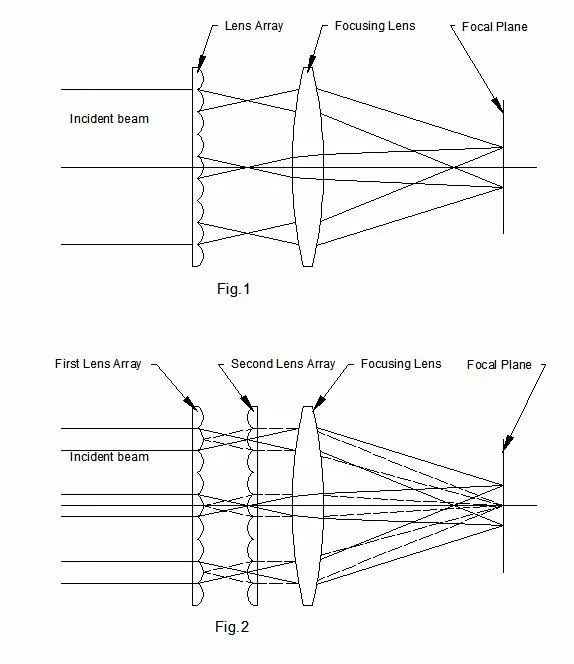
Single and dual lens array homogenizers
Homogenization is created by dividing the initial wave front into separate beams with each lens element and then focusing those new beams onto the target area with a focusing lens. (Fig.1). The separate beams are superimposed on the illumination plane focused with the focusing lens.
The shape of the illumination area is the same as that of the lens element aperture.
The quality of the uniformity is based on the number of lens elements contained in a lens array, which can be a limiting factor.
A disadvantage of using one lens array is that the sharpness of the illumination area edge can be reduced. The result is a blurred edge of the illumination spot, undesirable with some applications.
To eliminate this edge effect a second lens array can be used. (Fig.2)
One lens array is conjugated to another so that the focal points of one array coincide with the pupil surface of the next one, and the focal points of the second array coincide with the pupil surface of the first one. This setup improves edge sharpness but the arrays needs to be very accurately aligned with one another for it to work properly.
A second disadvantage stems from the diffraction between the lens element pairs resulting in different artifacts at the illumination area. Using a lens array with some irregularity in the lens elements’ packing can improve that but this solution is more challenging to manufacture.
An alternative approach is to use a diffraction optical element (DOE) instead of the lens elements.
Need assistance designing a custom illumination optic, lens or light pipe? Learn more about our design services here.
Light pipe homogenizing rod
A second method for homogenization is the use of a rod lens. In this layout, multiple reflections from the initial beam are mixed inside of the rod (or light pipe).
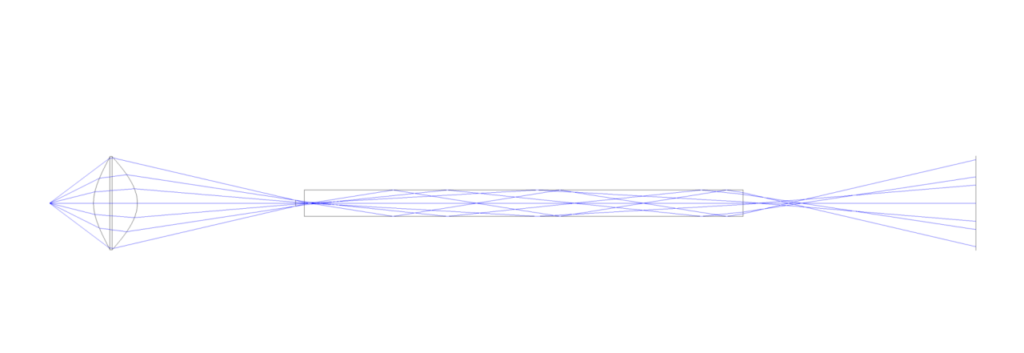
The length of the light pipe (homogenizing rod) should exceed several fold the laser beam cross section size, which dictates the longitudinal system size. In systems with space limitations, focusing the input beam into a light pipe with a focusing lens is an option for reducing the cross section size and longitudinal size.
Because the light beam has a lot of reflections in the light pipe, Fresnel reflection losses can be large. A light pipe which provides TIR reflection reduces reflection losses while a TIR angle limits the input beam’s numerical aperture (NA).
Summary of characteristics for light pipe vs lens array homogenizers
Characteristic | Lens array homogenizer | Light pipe homogenizer |
---|---|---|
Limit on beam diameter | no | yes |
Limit on NA | smaller | bigger |
Limit on size of source | bigger | smaller |
transmittance | Not critical | Critical with big NA |
Additional optics | Collimation lens | Focusing lens |
Alignment | Critical | Not critical |
Relative price | High | Low |
Applications of Laser Beam Homogenizers
Laser beam homogenizers find a myriad of applications across diverse fields where precise and uniform laser illumination is crucial. One prominent application lies within the realm of industrial manufacturing, particularly in processes like laser material processing and micromachining.
In these applications, uniform intensity distribution ensures consistent material removal rates and precise control over features such as cutting edges or engraved patterns. By employing laser beam homogenizers, manufacturers can enhance the quality and efficiency of their production processes, leading to improved product consistency and reduced waste.
Another significant area of application is in medical and biotechnological fields, where laser beams are utilized for procedures like laser surgery, tissue ablation, and microscopy. In surgical procedures, uniform laser illumination ensures precise tissue removal while minimizing collateral damage to surrounding areas.
Laser beam homogenization also plays a vital role in microscopy applications by providing uniform illumination across the sample, enabling clearer imaging and accurate analysis of biological specimens.
Moreover, laser beam homogenizers are instrumental in various scientific research endeavors, including optical trapping and manipulation, spectroscopy, and holography. In optical trapping, for instance, uniform laser intensity distribution facilitates stable trapping of microscopic particles or cells, enabling detailed studies of their properties and behaviors.
Similarly, in spectroscopic techniques like fluorescence microscopy or Raman spectroscopy, uniform illumination enhances signal-to-noise ratios and enables more accurate measurements of sample characteristics.
Furthermore, laser beam homogenizers find applications in emerging technologies such as additive manufacturing (3D printing), where precise control over laser energy distribution is critical for achieving uniform material deposition and layer fusion.
Additionally, homogenized laser beams are used in advanced imaging systems for lithography, photolithography, and projection displays, where uniform illumination is essential for producing high-resolution images with minimal distortion or artifacts.
Choosing the Right Homogenizer for Your Needs
Choosing the right homogenizer for your specific needs requires careful consideration of several factors to ensure optimal performance and efficiency. Here are key points to consider:
- Application Requirements: Start by identifying your application requirements. Different applications may necessitate specific beam profiles, intensity levels, or wavelength ranges. For instance, laser material processing may require a different homogenizer than laser microscopy or medical procedures.
- Beam Characteristics: Consider the characteristics of your input laser beam, such as its wavelength, power, divergence, and spatial profile. The homogenizer should be compatible with these beam characteristics to ensure effective beam shaping and uniformity.
- Homogenization Technique: Evaluate the various homogenization techniques available, such as diffractive optical elements (DOEs), microlens arrays, or refractive beam shapers. Each technique has its advantages and limitations, so choose one that best suits your application requirements and budget constraints.
- Spatial Uniformity: Assess the level of spatial uniformity required for your application. Some applications may tolerate slight variations in intensity across the beam profile, while others demand highly uniform illumination. Select a homogenizer capable of providing the desired level of spatial uniformity.
- Optical Efficiency: Consider the optical efficiency of the homogenizer, which refers to the percentage of input laser power that is effectively utilized for homogenization. Higher optical efficiency translates to less wasted energy and better overall performance.
- Flexibility and Customization: Determine whether the homogenizer offers flexibility and customization options to tailor the beam profile according to your specific needs. Some homogenizers may allow adjustment of parameters such as beam shape, size, or intensity distribution.
- Reliability and Durability: Choose a homogenizer from reputable manufacturers known for producing high-quality, reliable, and durable optical components. Ensuring the longevity and stability of the homogenizer is essential for long-term performance and cost-effectiveness.
- Cost Considerations: Evaluate the cost-effectiveness of different homogenizer options, taking into account not only the initial purchase price but also factors such as maintenance requirements, lifespan, and potential impact on overall system performance and productivity.
Innovations in Laser Beam Homogenization
In recent years, there have been notable innovations in laser beam homogenization aimed at addressing the evolving needs of various industries and research fields. Some key innovations include:
- Advanced Optical Designs: Engineers and researchers have developed sophisticated optical designs for laser beam homogenizers, leveraging techniques such as freeform optics, metasurfaces, and diffractive optical elements (DOEs). These designs allow for precise control over the spatial and spectral characteristics of the homogenized beam, enabling tailored solutions for specific applications.
- Adaptive Homogenization: Adaptive optics techniques have been integrated into laser beam homogenization systems to dynamically adjust the beam profile in real-time. By sensing and correcting for aberrations or disturbances in the optical path, adaptive homogenization ensures consistent performance even in challenging operating conditions, such as fluctuating environmental factors or changing input beam parameters.
- Multi-Wavelength Homogenization: With the increasing demand for multi-wavelength laser systems in various applications, there has been a focus on developing homogenization techniques capable of handling multiple wavelengths simultaneously. These innovations enable efficient homogenization of laser beams with different wavelengths, facilitating integration into complex optical setups and enabling multi-functional applications.
- Microscale Homogenization: In fields like microfluidics, biosensing, and microfabrication, there is a growing need for homogenization solutions tailored to microscale applications. Innovations in micro-optics and nanostructures have led to the development of compact, high-resolution homogenizers capable of producing uniform intensity distributions over small areas with high spatial precision.
- Nonlinear Optical Techniques: Nonlinear optical phenomena, such as beam shaping through nonlinear frequency conversion or Kerr-lens mode locking, have been explored for laser beam homogenization. These techniques offer unique advantages, such as wavelength versatility, high efficiency, and nonlinear control over the beam profile, opening up new possibilities for homogenization in specialized applications.
- Computational Homogenization: Computational methods, including numerical simulations and optimization algorithms, are increasingly being employed to design and optimize laser beam homogenization systems. By leveraging computational tools, researchers can explore a wide range of design parameters, predict performance characteristics, and iteratively refine homogenizer designs to achieve optimal results.
Conclusions
In conclusion, laser beam homogenization plays a critical role in numerous applications spanning industrial manufacturing, medical procedures, scientific research, and emerging technologies. Through innovative optical designs, adaptive techniques, and advancements in computational methods, significant progress has been made in achieving more uniform and precise laser illumination.
These innovations have enabled enhanced performance, efficiency, and versatility in laser-based processes, contributing to improved product quality, reliability, and cost-effectiveness across various industries.
Looking ahead, the future of laser beam homogenization holds promising opportunities for further advancements and applications. Continued research and development efforts are expected to focus on refining existing techniques, exploring new approaches, and addressing emerging challenges. Key areas of future innovation may include:
- Enhanced Adaptability: Further integration of adaptive optics and smart control algorithms to enable real-time optimization and adaptation of laser beam homogenization systems to changing operating conditions and application requirements.
- Ultrafast and High-Power Applications: Development of homogenization solutions capable of handling ultrafast laser pulses and high-power laser beams for applications such as femtosecond laser machining, laser-based particle acceleration, and high-energy physics experiments.
- Miniaturization and Integration: Advances in micro-optics, nanophotonics, and MEMS technology to enable compact, lightweight, and integrated homogenization solutions for portable devices, wearable technologies, and miniaturized optical systems.
- Multifunctional Homogenization: Exploration of multifunctional homogenization techniques capable of simultaneously achieving beam shaping, polarization control, wavelength conversion, and other functionalities to meet the diverse needs of complex optical systems and applications.
- Quantum Homogenization: Investigation of quantum-inspired homogenization techniques leveraging principles from quantum optics, quantum information processing, and quantum metrology to achieve unprecedented levels of precision, coherence, and control over laser beam properties.
FAQ: Understanding Laser Beam Homogenizers
What is a Laser Beam Homogenizer and its purpose?
A Laser Beam Homogenizer is a device used to make the intensity distribution of a laser beam uniform, enhancing beam quality for various applications.
How does a Laser Beam Homogenizer work?
It works by manipulating the laser light path through optical elements to distribute the beam’s intensity evenly.
What are the typical applications of Laser Beam Homogenizers?
They are widely used in areas like lithography, material processing, and biomedical applications where uniform light distribution is crucial.